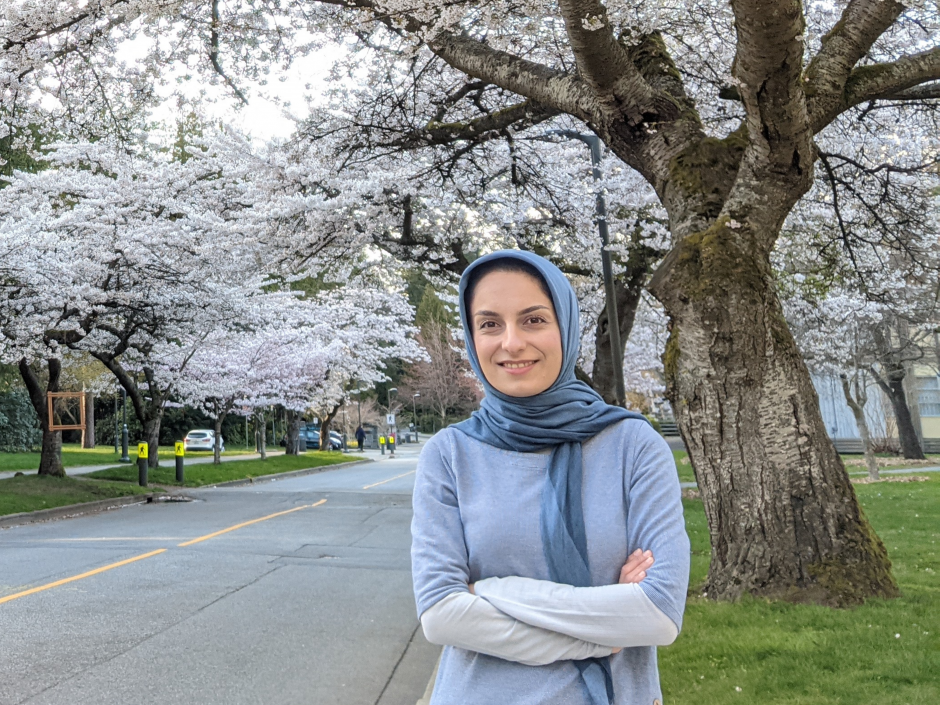
“Electrical and computer engineering is one of the most rapidly evolving fields of science, shaping people’s day-to-day lives.”
Melika Shahriari is a graduating PhD candidate in Electrical and Computer Engineering at UBC and TRIUMF, Canada’s particle accelerator centre. Melika received a MASc degree in Mechatronic Systems Engineering from Simon Fraser University, and her B.Sc. degree in Electrical Engineering from Sharif University of Technology, Iran.
Her research focuses on the control of the RF electromagnetic field of cavity resonators in particle accelerators. In this interview, Melika discusses her research, her experience at ECE, and her perspective on engineering- and shares highlights from her PhD thesis, Iterative Learning Control for Beam Loading Cancellation in Electron Linear Accelerators.
The draw of electrical and computer engineering
I’d always had this curiosity- I wanted to know what was going on in my computer or my phone, and I found that studying electrical engineering could answer some of my questions.
I think electrical and computer engineering is one of the most rapidly evolving fields of science. It shapes people’s day-to-day lives. Many of the biggest and most important modern technological advances- things like computers, cell phones, medical equipment and automotive systems- are owed to electrical and computer engineering.
Researching at TRIUMF
My research is based in TRIUMF, Canada’s particle accelerator centre, which is located at UBC. Particle accelerators are used to deliver beams of charged particles such as protons, electrons or heavy ions. These beams of accelerated particles are used for a variety of research topics- from astrophysics and the origin of our universe, to subatomic particles and particle physics.
My research involves cavity resonators, which are devices that generate an electric field to accelerate particles. I developed an iterative learning controller to predict and correct something called ‘cavity field error.’
Basically, if we want a high quality beam of particles, the electric field should be precisely controlled to ensure that all the particles receive the same energy. When the cavity accelerates a bunch of particles, its energy drops. This means there will be less energy available for the particles that arrive later, and there will be a lot of energy difference in the beam, which could lead to beam loss and instability. My research provides a solution by controlling the cavity field in a very short amount of time, which results in a uniformly accelerated beam.
These beams can be used for lots of different research activities. In TRIUMF, for example, apart from research in physics, the proton beam is used for treating eye tumours, and the Positron Emission Tomography program develops novel radioactor for medical imaging, which can be used for researching Parkinson’s disease.
I’d spent almost a year developing the control system on a new digital field-programmable gate array (FPGA) board, which is an integrated circuit that the user can configure and change its functionality. But, due to COVID-19, we never got to commission the new board, since we lost access to the lab.
I managed to do some tests with a different implementation of my controller on the current control system in the TRIUMF electron linear accelerator just before the pandemic, but because of the pandemic I didn’t get to compare those results with the controller on the FPGA board.
Work after graduation
I’ll be joining the Intel programmable solutions group in Toronto after I graduate. Intel is one of the biggest field-programmable gate array (FPGA) manufacturers in the world. FPGAs are usually used for applications that require high speed, where conventional processors can’t meet the timing requirements.
I had to learn FPGA for my research, so I was using the product as a user. I’m very excited to join the developer-side now! One interesting fact is that the team manager of the group I’m joining is actually also a PhD graduate of UBC ECE.
Insights on her time with ECE at UBC
With my research, I really enjoyed getting to meet and work with so many brilliant minds, and having the luxury of working in a great lab with advanced technology. Conducting research in a multidisciplinary field has helped me learn a lot, especially from physicists.
My advice for others entering this field is to not underestimate the importance of connecting with people, be it other fellow students, professors, grads or people in the industry. Sometimes when you have a heavy workload, there’s a tendency to isolate yourself to get your work done. But I think now that the human connection is largely missing from our lives due to COVID-19, it’s easier to realize its importance.
Highlights from Melika Shahriari’s thesis: Iterative Learning Control for Beam Loading Cancellation in Electron Linear Accelerators
Radiofrequency (RF) cavities are metal structures that contain a standing wave electromagnetic field. The electric component of the field is used for particle acceleration. If a particle with charge q passes through the electromagnetic filed, the total force it experiences is given by Lorentz law as
F = q(E + v × B),
where E is the electric field, B is the magnetic field and v is the velocity of the particle.
A nine-cell superconducting cavity resonator used in TRIUMF electron linear accelerator (e-linac) is shown in Figure 1, and Figure 2 shows the field distribution in a single cell.
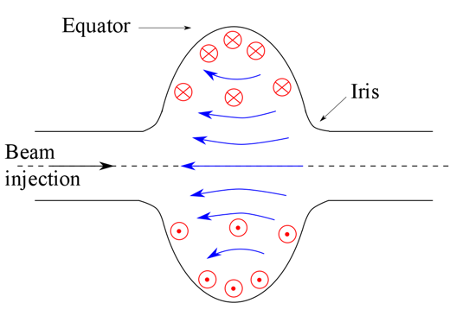
There are multiple phases of operation for a cavity resonator illustrated in Figure 3. In the filling phase, the generator stores energy into the cavity, and the cavity voltage increases with a time constant and reaches the desired value, like charging a capacitor. In the flat-top phase, when there is no beam, the generator provides energy to compensate for ohmic losses, which is pretty small in superconducting cavities. When beam is injected, energy is transferred from the cavity to the beam, and the cavity voltage drops, like discharging of a capacitor. This is called the beam loading effect. It is desired to cancel this effect as fast as possible and maintain a constant accelerating field.
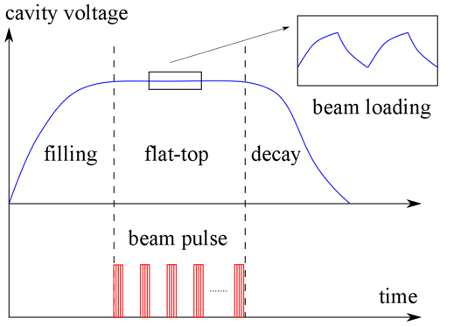
Feedback control is typically not fast enough to reach the desired response time. Therefore, feedforward control is often used in parallel to a feedback controller. The feedforward controller could be a constant gain. For complete beam loading cancellation, the gain should be equal to the voltage drop due to beam loading. An exact prediction of the beam current and the resulting voltage drop is required for effective beam loading cancellation. If there is an offset error due to wrong approximation, the error repeats at every pulse.
In this research, iterative learning control (ILC) is used in parallel to a constant-gain feedforward controller to predict the beam loading effect and cancel it by learning from the previous beam pulses. ILC is an open loop control strategy for systems that perform the same task repetitively. It improves the system performance by learning from the previous iterations.
The proposed iterative learning control was designed and tested on TRIUMF e-linac injector cavity (EINJ). The results are shown in Figure 4. The blue signal shows the cavity voltage when there is only the feedback controller in the loop. The beam loading effect can be clearly observed as a decrease in cavity voltage. The green signal shows the cavity voltage with the manual constant-gain feedforward controller. Beam loading cancellation is slightly improved. The best result is achieved when the ILC is on, shown in red. The beam loading effect is almost fully cancelled. Figure 5 shows the result from beam position monitor (BPM). BPM measure the energy of different electrons in the beam by measuring their position. In the ideal case, the BPM signal is flat, indicating that all the electrons have the same energy. In Figure 5, the blue curve shows the BPM data for the case where there is only a feedback controller. Due to lack of beam loading cancellation, the beam energy drops as time goes on. Turning the manual feedforward on results in the red curve, which is slightly better but still the beam energy decreases. The most stable beam energy is achieved with the ILC, shown with green, where the energy is mostly constant throughout the pulse.
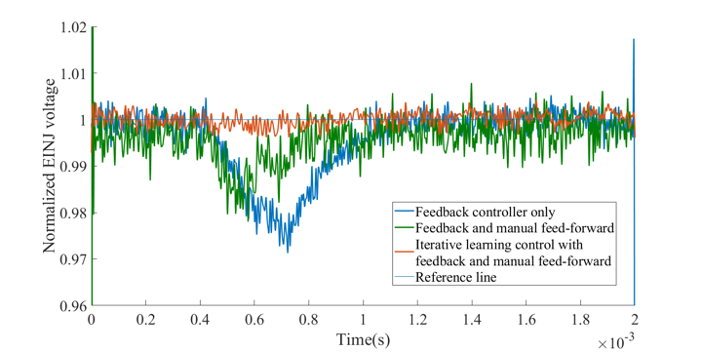
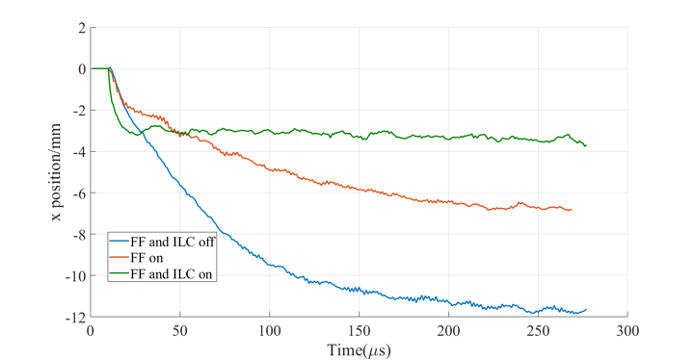
If you want to connect with Melika about her research, she can be reached at shahriari.melika@gmail.com.
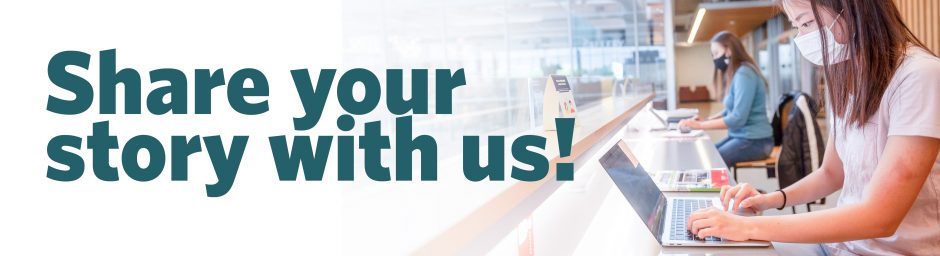